
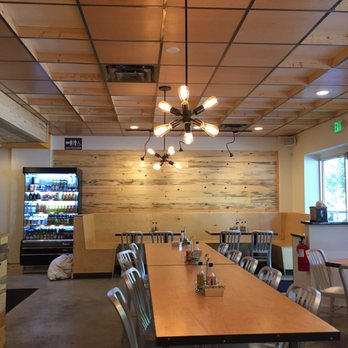
Three previous approaches have been used to address issues of specimens thicker than the ~50 µm limit (the typical depth of focus limit for gallium FIB beams) 9 prior to FIB-milling lamellae for imaging in a transmission electron microscope (TEM). However, the broad application of conventional cryo-FIB/SEM with reasonable throughput is often challenging for thick specimens with vitrification issues and specimens with dimensions smaller than about 2 µm which adopt a preferred orientation on the grid.
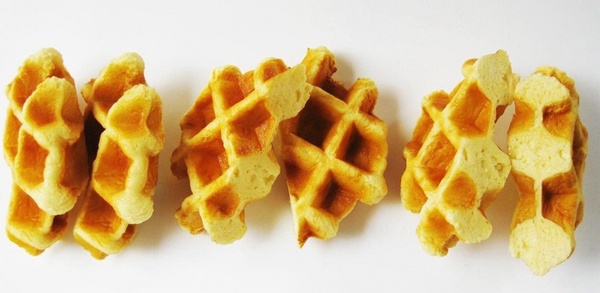
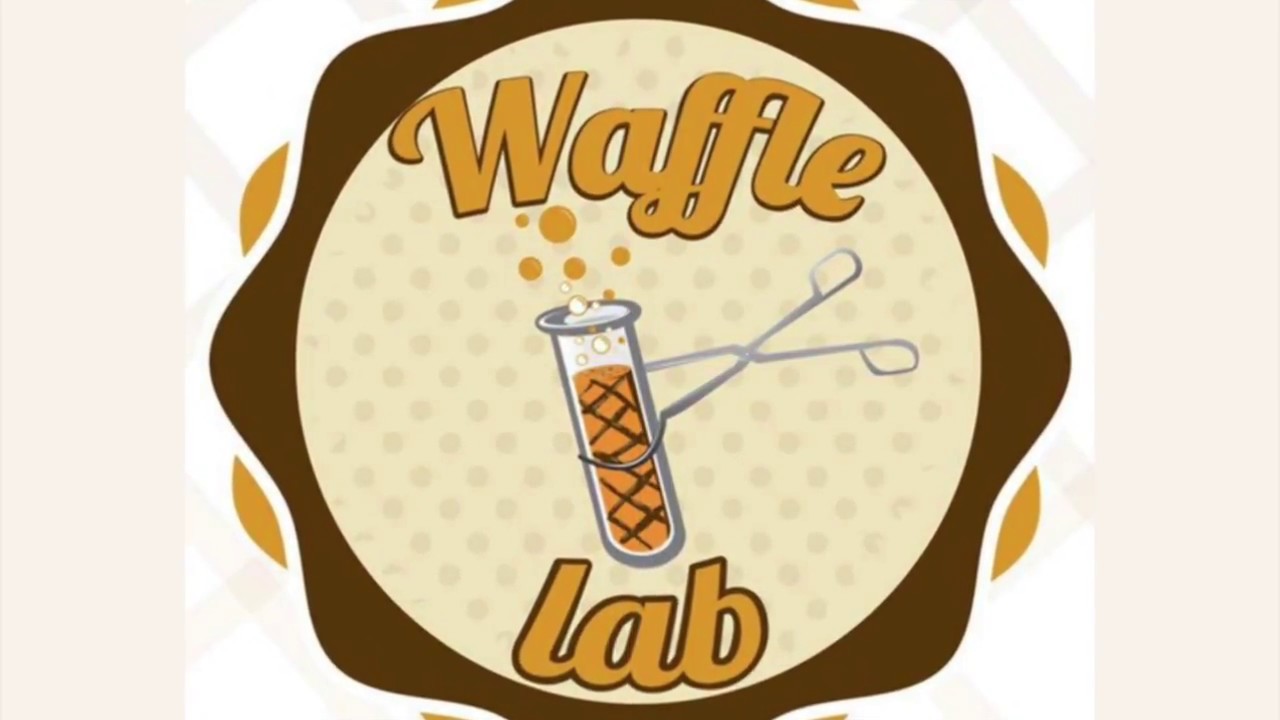
Methods for promoting cell adhesion onto grids have been developed 6– 8. In some instances, cryo-FIB/SEM followed by cryo-ET (cryo-FIB/SEM-ET) and sub-tomogram processing has produced in-situ 3D structures of molecular complexes at resolutions on the order of 1 nm 2– 5. We propose the Waffle Method as a way to achieve many advantages of cryo-liftout on the specimen grid while avoiding the long, challenging, and technically-demanding process required for cryo-liftout.Ĭryo-focused ion beam milling scanning electron microscopy (cryo-FIB/SEM) 1 of intact cells plunge frozen onto EM grids (conventional cryo-FIB/SEM) followed by cryo-electron tomography (cryo-ET) is developing as a fruitful sample preparation and analysis method for structural studies of cells thinner than about 10 µm. We also present a unique and critical stress-relief gap designed specifically for waffled lamellae. We demonstrate the broadness of the Waffle Method by applying it to three additional cellular samples and a single particle sample using a variety of cryo-FIB-milling hardware, with manual and automated approaches. We illustrate the mitigation of these challenges by applying the Waffle Method and cryo-ET to reveal the macrostructure of the polar tube in microsporidian spores in multiple complementary orientations, which was previously not possible due to preferred orientation. Here we present a general approach called the ‘Waffle Method’ which leverages high-pressure freezing to address these challenges.
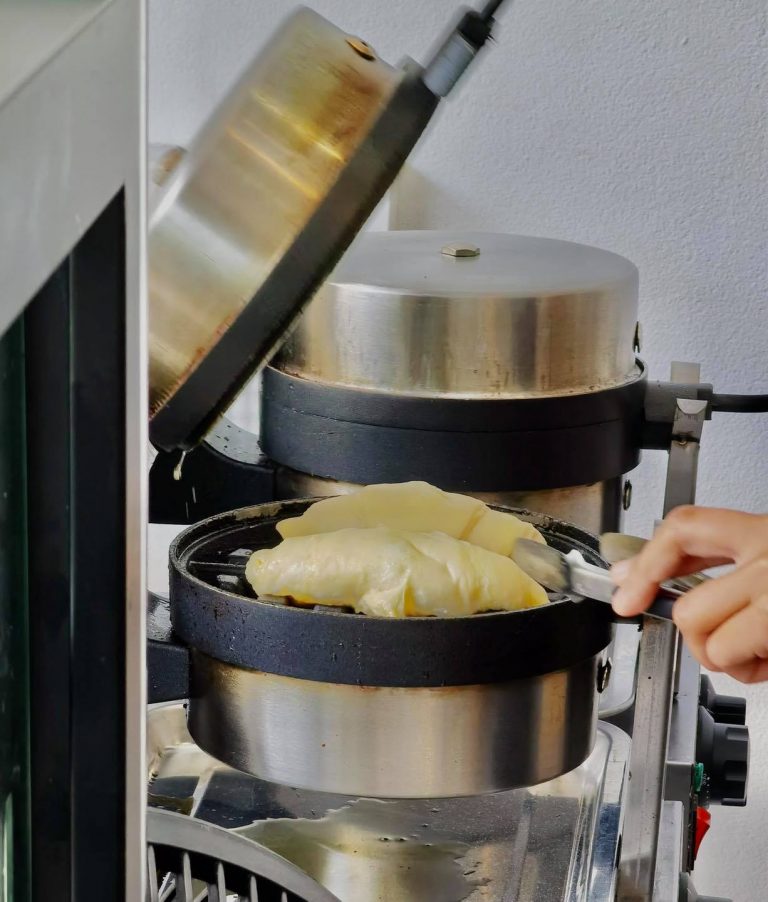
However, challenges remain in conventional cryo-FIB/SEM workflows, including milling thick specimens with vitrification issues, specimens with preferred orientation, low-throughput when milling small and/or low concentration specimens, and specimens that distribute poorly across grid squares. Cryo-FIB/SEM combined with cryo-ET has emerged from within the field of cryo-EM as the method for obtaining the highest resolution structural information of complex biological samples in-situ in native and non-native environments.
